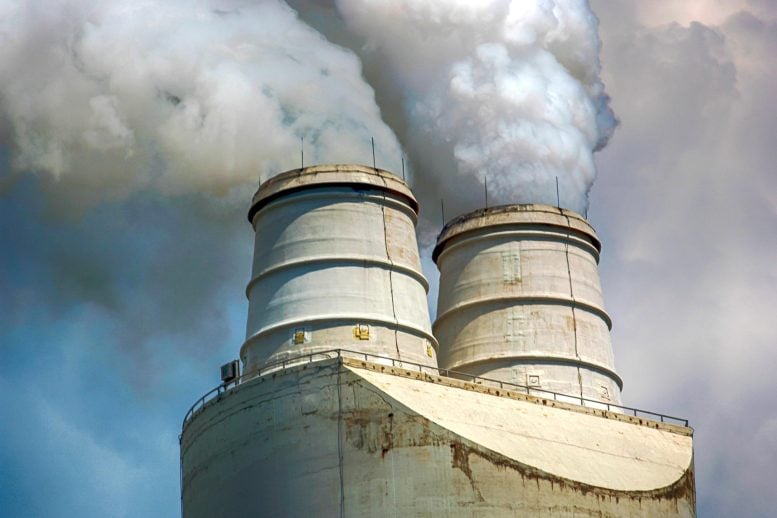
University of Toronto engineering researchers have developed a new catalyst that efficiently converts captured carbon into valuable products such as ethylene and ethanol, even in the presence of sulfur oxide contaminants. The breakthrough offers a more economically viable method for carbon capture and recovery, and could revolutionize industries such as steelmaking and cement by allowing them to more efficiently convert CO2 from waste streams.
An electrochemical catalyst for converting CO2 into valuable products can withstand an impurity that plagues current versions.
A new catalyst improves the conversion of captured carbon into commercial products, while maintaining high efficiency despite sulfur oxide impurities. This innovation could significantly reduce the costs and energy requirements of carbon capture technologies, impacting heavy industries.
A newly designed catalyst, created by University of Toronto engineering researchers, efficiently converts captured carbon into valuable products, even in the presence of a contaminant that degrades the performance of current versions.
This discovery represents an important step towards more economically favorable carbon capture and storage techniques that could be added to existing industrial processes.
Advances in carbon conversion technologies
“Today we have more and better options for low-carbon electricity generation than ever before,” says Professor David Sinton (MIE), lead author of a paper published in Natural energy July 4th which describes the new catalyst.
“But other sectors of the economy will be harder to decarbonise: for example, steel and cement production. To help these industries, we need to invent cost-effective ways to capture and valorise the carbon contained in their waste streams.”
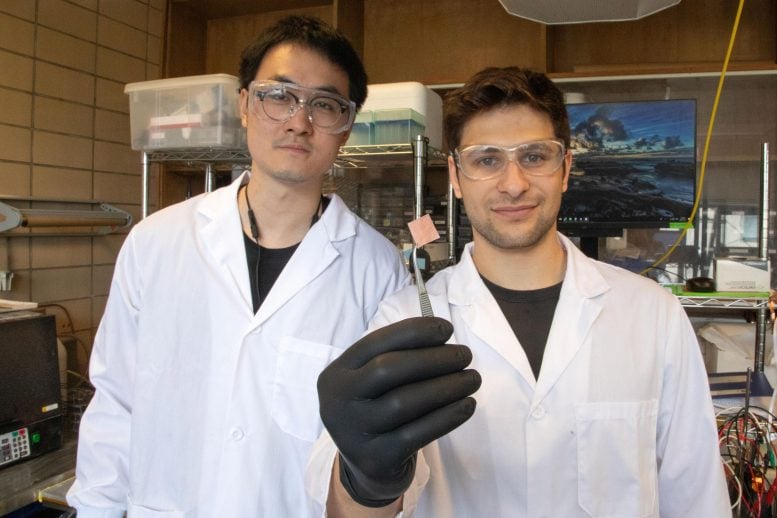
University of Toronto engineering PhD students Rui Kai (Ray) Miao (left) and Panos Papangelakis (right) hold a new catalyst they designed to convert captured CO2 gas into valuable products. Their version works well even in the presence of sulfur dioxide, a contaminant that poisons other catalysts. Credit: Tyler Irving / University of Toronto Engineering
Use of electrolyzer in carbon transformation
Sinton and his team use devices called electrolyzers to convert CO2 and electricity into products such as ethylene and ethanol. These carbon-based molecules can be sold as fuels or used as chemical feedstocks for making everyday items such as plastic.
Inside the electrolyser, the conversion reaction occurs when three elements – CO2 gas, electrons and a liquid water-based electrolyte – come together on the surface of a solid catalyst.
The catalyst is often made of copper, but it can also contain other metals or organic compounds that can further improve the system. Its function is to speed up the reaction and minimize the creation of unwanted side products, such as hydrogen gas, which reduce the efficiency of the overall process.
Meeting the challenges of catalyst efficiency
While many teams around the world have produced very successful catalysts, almost all of them are designed to run on a pure CO2 feedstock. But if the carbon in question comes from industrial smokestacks, the feedstock may be anything but pure.
“Catalyst designers generally don’t like working with impurities, and for good reason,” says Panos Papangelakis, a doctoral student in mechanical engineering and one of five co-lead authors of the new paper.
“Sulfur oxides, such as SO2, poison the catalyst by binding to the surface. This leaves fewer sites for CO2 to react and also causes unwanted chemicals to form.
“It happens very quickly: while some catalysts can last hundreds of hours with a pure charge, if you introduce these impurities, within minutes their efficiency can be reduced to 5%.”
While there are well-established methods for removing impurities from CO2-rich exhaust gases before introducing them into the electrolyser, they are time-consuming, energy-intensive and increase the cost of carbon capture and recovery. Moreover, in the case of SO2, even a small amount can be a big problem.
“Even if you reduce your exhaust gases to less than 10 parts per million, or 0.001 percent of the load, the catalyst can still be poisoned in less than 2 hours,” Papangelakis says.
Innovations in catalyst design
In the paper, the team describes how they designed a more resilient catalyst that could withstand SO2 by making two key modifications to a typical copper-based catalyst.
On one side, they added a thin layer of polytetrafluoroethylene, also known as Teflon. This non-stick material changes the chemistry on the surface of the catalyst, preventing the reactions that allow SO2 poisoning.
On the other side, they added a layer of Nafion, an electrically conductive polymer often used in fuel cells. This complex porous material contains hydrophilic areas, which attract water, as well as other hydrophobic areas, which repel water. This structure makes it difficult for SO2 to reach the surface of the catalyst.
Performance in adverse conditions
The team then fed this catalyst with a mixture of CO2 and SO2, the latter having a concentration of about 400 parts per million, which is typical of an industrial waste stream. Even under these harsh conditions, the new catalyst performed well.
“In the paper, we report a Faraday efficiency — a measure of how many electrons end up in the desired products — of 50 percent, which we were able to maintain for 150 hours,” Papangelakis says.
“Some catalysts may be more efficient initially, maybe 75 or 80 percent. But if you expose them to SO2, within minutes or at most a few hours, their efficiency drops to almost zero. We were able to withstand that.”
Future directions and implications
Papangelakis says that because his team’s approach doesn’t affect the composition of the catalyst itself, it should be applicable on a large scale. In other words, teams that have already perfected high-performance catalysts should be able to use similar coatings to provide resistance to sulfur oxide poisoning.
Although sulfur oxides are the most difficult impurity to remove from conventional waste streams, they are not the only ones, and the team then turns to the full range of chemical contaminants.
“There are many other impurities to consider, such as nitrogen oxides, oxygen, etc.,” Papangelakis says.
“The fact that this approach works so well for sulfur oxides is very promising. Before this work, it was taken for granted that you had to remove the impurities before you could valorize the CO2. What we’ve shown is that there may be another way to treat them, which opens up a lot of new possibilities.”
Reference: “Improving SO2 Tolerance of CO2 Reduction Electrocatalysts Using a Polymer/Catalyst/Ionomer Heterojunction Design” by Panagiotis Papangelakis, Rui Kai Miao, Ruihu Lu, Hanqi Liu, Xi Wang, Adnan Ozden, Shijie Liu, Ning Sun, Colin P. O’Brien, Yongfeng Hu, Mohsen Shakouri, Qunfeng Xiao, Mengsha Li, Behrooz Khatir, Jianan Erick Huang, Yakun Wang, Yurou Céline Xiao, Feng Li, Ali Shayesteh Zeraati, Qiang Zhang, Pengyu Liu, Kevin Golovin, Jane Y. Howe, Hongyan Liang, Ziyun Wang, Jun Li, Edward H. Sargent, and David Sinton, July 4, 2024, Natural energy.
DOI: 10.1038/s41560-024-01577-9