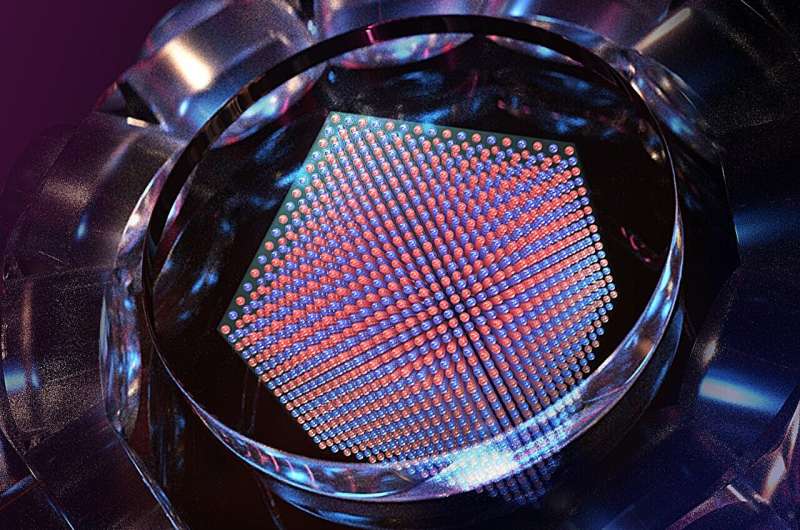
This image shows the ultracold atomic quantum simulator. The red and blue balls represent the fermionic atoms with spins up and down, respectively, arranged in a staggered pattern in 3D space, forming the antiferromagnetic crystal. The glass cell provides the ultrahigh vacuum environment for the ultracold atoms. Credit: Chen Lei
In a study published in NatureA research team has observed for the first time the antiferromagnetic phase transition within a large-scale quantum simulator of the fermionic Hubbard model (FHM).
This study highlights the advantages of quantum simulation. It marks an important first step toward obtaining the low-temperature phase diagram of FHM and understanding the role of quantum magnetism in the mechanism of high-temperature superconductivity. The team was led by Professor Pan Jianwei, Professor Chen Yuao, and Professor Yao Xingcan from the University of Science and Technology of China (USTC), Chinese Academy of Sciences.
Strongly correlated quantum materials, such as high-temperature superconductors, are of scientific importance and have potential economic benefits. However, the physical mechanisms underlying these materials remain unclear, posing challenges for their preparation and large-scale application.
The FHM, a simplified representation of electron behaviors in a lattice, captures a wide range of physics related to strong correlations, similar to those observed in quantum materials, and is therefore believed to potentially offer solutions to understanding the mechanism of high-temperature superconductivity.
The study of the FHM is fraught with challenges. There is no exact analytical solution for this model in two and three dimensions, and due to the high computational complexity, even the most advanced numerical methods can only explore limited parameter spaces. Moreover, theoretical studies suggest that even a universal digital quantum computer would struggle to solve this model accurately.
It is widely accepted that quantum simulation, using ultracold fermionic atoms in optical lattices, may be the key to mapping the low-temperature phase diagram of the FHM. To this end, realizing the antiferromagnetic phase transition and reaching the half-filling FHM ground state are the most important steps.
Such an achievement would validate two key capabilities of the quantum simulator: establishing a large-scale spatially homogeneous optical lattice for uniform Hubbard parameters, and maintaining a system temperature significantly below the Néel temperature, the antiferromagnetic phase transition temperature, both essential for exploring the role of quantum magnetic fluctuations in the mechanism of high-temperature superconductivity.
However, the difficulty of cooling fermionic atoms and the inhomogeneity introduced by a standard Gaussian profile lattice laser have hampered the realization of the antiferromagnetic phase transition in previous quantum simulation experiments. To address these challenges, the team, building on their previous achievements in preparing and studying strongly interacting homogeneous Fermi gases in a box potential, developed an advanced quantum simulator by combining the generation of a low-temperature homogeneous Fermi gas in a box trap with the demonstration of a flat-top optical lattice with uniform site potentials.
This quantum simulator contains about 800,000 lattice sites, about four orders of magnitude larger than current experiments with several dozen sites, and features uniform Hamiltonian parameters with temperatures significantly below the Néel temperature.
Using this setup, the team fine-tuned the interaction strength, temperature, and doping concentration to approach their respective critical values, and directly observed conclusive evidence for the antiferromagnetic phase transition, i.e., the power-law divergence of the spin structure factors, with a critical exponent of 1.396 from Heisenberg universality.
This work advances the understanding of quantum magnetism and lays the foundation for further solving the FHM and obtaining its phase diagram at low temperatures. Notably, experimental results deviating from the half-filling condition have already exceeded the capabilities of current classical computing, demonstrating the advantages of quantum simulation for solving key scientific problems.
More information:
Jian-Wei Pan, Antiferromagnetic phase transition in a 3D fermionic Hubbard model, Nature (2024). DOI: 10.1038/s41586-024-07689-2. www.nature.com/articles/s41586-024-07689-2
Provided by Chinese Academy of Sciences
Quote: Fermionic Hubbard Quantum Simulator Observes Antiferromagnetic Phase Transition (July 10, 2024) Retrieved July 11, 2024 from https://phys.org/news/2024-07-fermionic-hubbard-quantum-simulator-antiferromagnetic.html
This document is subject to copyright. Apart from any fair dealing for the purpose of private study or research, no part may be reproduced without written permission. The content is provided for informational purposes only.