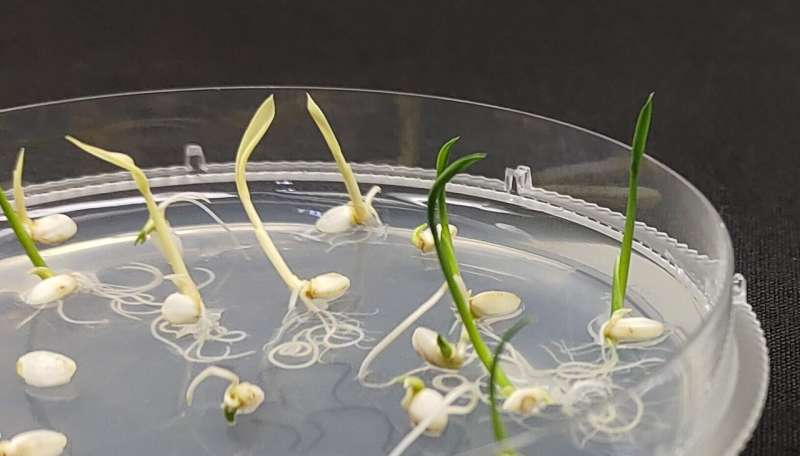
Albino rice plants created with TnpB by disrupting the gene responsible for producing green color. Credit: Kutubuddin Molla and Subhasis Karmakar
Genome editing is one of the most transformative scientific advances of our time. It allows us to delve into the very code of life and make precise changes. Imagine being able to rewrite the genetic instructions that determine almost everything about an organism: how it looks, how it behaves, how it interacts with its environment, and what unique characteristics it has. That’s the power of genome editing.
We use genome editing tools to modify the genetic sequences of microbes, animals, and plants. Our goal? To develop the desired traits and eliminate the unwanted ones. The impact of this technology has been felt in the fields of biotechnology, human therapeutics, and agriculture, bringing rapid advances and solutions.
The most commonly used proteins in genome editing are Cas9 and Cas12a. These proteins are like the scissors of the genetic world, allowing us to cut and edit DNA. However, they are quite large, consisting of 1,000 to 1,350 amino acids. Advanced editing technologies like base editing and core editing require additional proteins to be fused to Cas9 and Cas12a, making them even larger. This size poses a challenge in efficiently introducing these proteins into cells, where the genetic material resides.
But now we have an exciting development: a miniature alternative that promises to overcome this limitation. In our recent article in the Journal of Plant BiotechnologyWe presented TnpB, a smaller but highly efficient next-generation tool for plant genome editing.
TnpBs are tiny ancestors of the Cas12 nuclease
TnpB proteins are RNA-guided transposon-associated nucleases. They are considered the evolutionary ancestors of Cas12 nucleases. Although TnpB is functionally similar to Cas12a, it is much more compact, with a total amino acid count of between 350 and 500. To put this in perspective, TnpB is one-third the size of Cas9 and Cas12a. If Cas9 and Cas12a are like footballs, TnpB are like baseballs.
We have developed a hypercompact genome editor using the TnpB nuclease from Deinococcus radiodurans. This bacterium is known for its ability to survive extreme environments and its remarkable resistance to radiation. Our TnpB, from D. radiodurans, has only 408 amino acids.
A short RNA serves as a guide for TnpB, directing it to the target DNA sequence. Specified by this RNA, TnpB binds to the target and cuts both strands of DNA. When the broken ends are resealed by the cell, inadvertent insertions or deletions of DNA letters can occur. These insertions or deletions result in altered genetic sequences.
There is an additional level of specificity: the target sequence must be adjacent to a TAM (Transposon Associated Motif) sequence. This TAM sequence is analogous to the PAM sequence of Cas9 and Cas12. For D. radiodurans TnpB, the specific TAM is TTGAT, which must be present upstream of the target sequence. In this sense, TnpB can access genomic loci that Cas9 cannot reach.
Repurposing TnpB for plant genome editing
We first optimized the TnpB protein sequence at the codon level to develop a genome editor for plant systems. We also optimized the combinations of regulatory elements to produce enough guide RNA for high-efficiency plant genome editing. By testing four different versions of genome editing vector systems in rice protoplasts, we identified the most efficient version.
Rice is a monocot, and systems that work well in monocots may not work as well in dicots. Therefore, we generated dicot-specific TnpB vectors and demonstrated successful editing in Arabidopsis. Interestingly, we observed that deletions occurred primarily at target loci in both rice and Arabidopsis. This makes TnpB capable of efficiently disrupting gene functions. TnpB could now be used to introduce genetic mutations to disrupt unwanted genes to eliminate antinutrients, improve nutrient content, resistance to biotic and abiotic stress, and more.
A dead TnpB for gene activation and exchange of single DNA letters
While TnpB in its native form acts as programmable scissors, it can also be adapted to recruit factors that activate genes. By inactivating its cutting ability, we developed deactivated TnpB (dTnpB). dTnpB retains its ability to bind to target DNA specified by the guide RNA. We then fused dTnpB with additional cargo proteins to funnel them to target genes, making these genes more active. This activation tool can boost gene function, paving the way for creating better crops in the future.
Similarly, we fused another cargo protein with dTnpB to develop a tool that can swap one DNA letter for another. This precise tool will enable innovation in crops by modifying the genetic code with a resolution of a single letter.
We are exploiting this miniature genome editor to engineer rice plants with improved yields and increased climate resilience. Our research highlights that TnpB is a highly versatile and promising tool for plant genome engineering. We hope that plant biologists, biotechnologists, and breeders will adopt TnpB for use in a variety of crops.
This article is part of Science X Dialog, where researchers can present the results of their published research papers. Visit this page for information about Science X Dialog and how to participate.
More information:
Subhasis Karmakar et al, A miniature alternative to Cas9 and Cas12: transposon-associated TnpB mediates targeted genome editing in plants, Journal of Plant Biotechnology (2024). DOI: 10.1111/pbi.14416
Dr. Kutubuddin Molla is an agricultural biotechnology scientist at ICAR-National Rice Research Institute (NRRI) in Cuttack, India. He received his PhD from the University of Calcutta, Kolkata. Dr. Molla conducted postdoctoral research at Pennsylvania State University under Fulbright Scholarship.
Dr. Molla’s research focuses on precise genome editing, using CRISPR-Cas and other advanced techniques for crop improvement. His lab at NRRI is dedicated to developing new genome editing tools and applying them to improve crop performance.
Quote:Tiny TnpB: Next-generation genome editing tool for plants unveiled (July 10, 2024) retrieved July 10, 2024 from https://phys.org/news/2024-07-tiny-tnpb-generation-genome-tool.html
This document is subject to copyright. Apart from any fair dealing for the purpose of private study or research, no part may be reproduced without written permission. The content is provided for informational purposes only.