Every year, approximately 1.8 million people worldwide are bitten by snakes. Of these, up to 138,000 die and another 400,000 are left with permanent scarring and disability.
Many cobras have venoms that damage tissue and cannot be treated with current antivenoms. We have discovered that cheap and readily available anticoagulant drugs can be repurposed as antidotes for these venoms.
Using CRISPR gene-editing technology, we learned how these venoms attack our cells and discovered that a common class of drugs called heparinoids can protect tissues from the venom. Our research is published today in Scientific translational medicine.
Snake bites are a serious problem
Snake venoms are made up of many different compounds. They typically target the heart, nervous system, or tissues at the site of exposure (such as skin and muscles).
Most research on snakebites naturally focuses on the most deadly venoms. Therefore, venoms that are less deadly but still cause long-term problems, such as cobra venom, have received less attention.
In areas where cobras live, severe snakebites can have devastating effects such as amputation, leading to life-changing injuries and loss of livelihoods. The World Health Organization has classified snakebites as a “category A” neglected tropical disease and hopes to halve the number of snakebites by 2030.
The only current treatments for snakebites are antivenoms, which are made by exposing nonhuman animals to small amounts of venom and harvesting the antibodies they produce in response.
Antivenoms save lives, but they have several drawbacks. Each is specific to one or more snake species, they are prohibitively expensive (when available), they must be kept cold, and they must be administered by injection in a hospital.
Additionally, antivenoms cannot prevent local tissue damage. This is mainly because the antibodies they contain are too large to reach peripheral tissues, such as a limb.
How Cobra Venom Kills Cells
Our team – from the University of Sydney in Australia, the Liverpool School of Tropical Medicine in the UK and the Instituto Clodomiro Picado in Costa Rica – set out to find other options for treating snakebites.
We first wanted to understand how these venoms work. We started with cobras, which are found throughout Africa and South Asia.
We took venom from the African spitting cobra, which is known to cause tissue damage, and performed what’s called a whole-genome CRISPR screen.
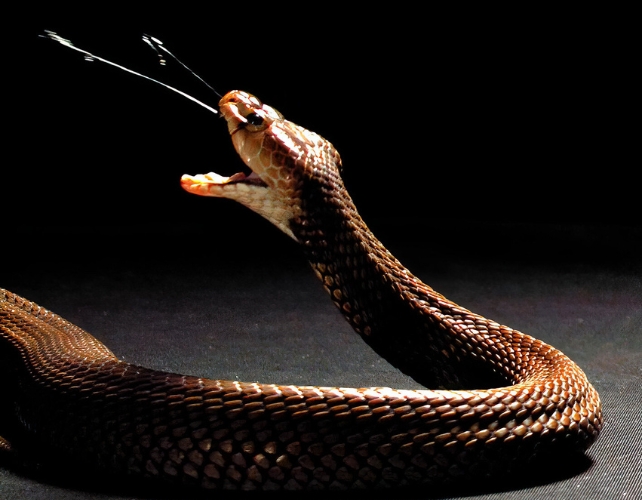
We took a large mixture of human cells and used CRISPR gene editing technology to disable a different gene from the entire human genome in each cell. CRISPR technology uses a special enzyme to delete or modify specific parts of DNA in a cell.
We then exposed all the cells to the cobra venom and observed which ones survived and which ones died.
The cells that survived must have lacked what the venom needed to harm us, so we were able to quickly identify what those characteristics were.
We discovered that several cobra venoms require specific enzymes to kill human cells. These enzymes are responsible for producing long sugar molecules called heparan and heparin sulfate.
Heparan sulfate is found on the surface of human and animal cells. Heparin sulfate is released by our cells when our immune system responds to a threat.
The importance of these molecules makes intuitive sense. Snake venoms evolved along with their targets, and heparan and heparin have changed very little over the course of evolution. So the venoms have hijacked something common to animal physiology to cause harm.
How heparin-based decoys reduce tissue damage
Heparin has been used as an anticoagulant drug for nearly 100 years.
We tested this drug on human cells to see if flooding the system with free heparin could be used as a decoy target for the venom. Surprisingly, it worked and the venoms no longer caused cell death, even when heparin was added to the cells after the venom.
We also tested heparin against the venom of Asian cobras, a distantly related species, and it had the same protective effect. We also showed that injecting a smaller synthetic version of heparin, called tinzaparin, could reduce tissue damage in mice that had been given an artificial “snake bite.”
To understand how heparin blocked the venom, we separated the venom into its major components. We found that heparin inhibits “three-finger cytotoxic toxins,” which are a major cause of tissue damage. Until now, there were no drugs known to work against these toxins.
The next step will be to test the effects of heparin on people.
Snakebite treatment cheaper and more accessible
Our goal is to make a snakebite treatment device containing heparin-like drugs called heparinoids, which would be similar to the epinephrine injectors EpiPens often carried by people at risk of severe allergic reactions. These devices could be distributed to people at high risk of cobra bites.
Heparinoids are already essential, inexpensive drugs used to prevent blood clots. The U.S. Food and Drug Administration has approved them for self-administration in humans, which could reduce the time it takes to get a drug to market.
Heparinoids are also stable at room temperature, meaning the drugs can be more accessible in remote areas and delivered more quickly in the field.
Other studies have also confirmed the usefulness of repurposing drugs to treat snakebites. These drug combinations could mark the beginning of a new era of snake venom treatment that does not rely solely on expensive antivenoms.
Our lab has already used CRISPR screening to study box jellyfish venom, and we are currently studying other venoms closer to home, from bluebottles to black snakes. Our screening technique allows us to uncover a wealth of information about a venom.
It’s still early to tell, but we’re seeing that many venoms attach to our cells by binding to overlapping targets. This research feeds into the larger goal of creating universal, broad-based antidotes for venoms.
Tian Du, PhD student in venom genomics, University of Sydney and Greg Neely, Professor of Functional Genomics, University of Sydney
This article is republished from The Conversation under a Creative Commons license. Read the original article.