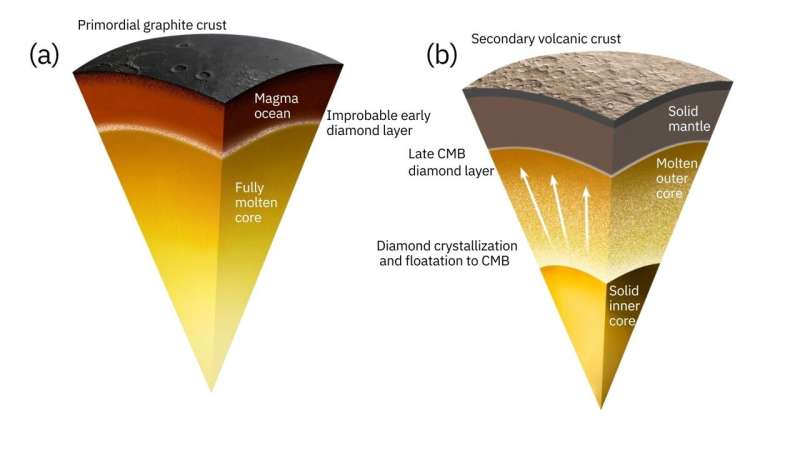
Proposed scenario for diamond formation at the core-mantle boundary of Mercury. (a) Crystallization of the carbon-saturated silicate magma ocean and potential, but unlikely, early production of diamond at its base. Graphite was the major phase to form in the magma ocean and accumulated at the surface to form a primordial graphite crust. (b) During crystallization of the inner core, diamond dissolved and floated to the core-mantle boundary. Such a late diamond layer would have continued to grow throughout core crystallization. Credit: Dr. Yanhao Lin and Dr. Bernard Charlier.
A recent study in Nature Communications Studies by Chinese and Belgian scientists suggest that Mercury’s core-mantle boundary (CMB) includes a diamond layer, potentially up to 18 kilometres thick, deep inside the planet.
Mercury, the smallest and innermost planet in our solar system, has long intrigued scientists because of its remarkably dark surface and the high density of its core. Previous missions, such as NASA’s MESSENGER spacecraft, have revealed that Mercury’s surface contains significant amounts of graphite, a form of carbon.
The researchers concluded that the planet’s early history involved a carbon-rich magma ocean. Phys.org spoke with one of the study’s co-authors, Dr. Yanhao Lin of the Advanced Research Center for High-Pressure Science and Technology in Beijing.
“Many years ago, I noticed that Mercury’s extremely high carbon content could have important consequences. This made me realize that something special had probably happened inside its body,” Dr. Lin said.
What we know about Mercury
The most detailed information about Mercury comes from NASA’s MESSENGER and Mariner 10 missions.
Previous observations by the MESSENGER spacecraft revealed that Mercury’s surface was unusually dark due to the widespread presence of graphite.
The abundance of carbon on the surface is thought to come from an ancient layer of graphite that floated to the surface a very long time ago. This suggests that Mercury once had a molten surface layer or magma ocean containing a significant amount of carbon.
Over time, as the planet cooled and solidified, this carbon formed a graphite crust on the surface.
However, the researchers question the hypothesis that graphite was the only stable phase containing carbon during the crystallization of Mercury’s magma ocean, at a time when the planet’s mantle (intermediate layer) cooled and solidified.
Early assumptions about the graphite crust were based on predictions of lower temperatures and pressures for the CMB. But more recent studies suggest that the CMB is deeper than previously thought, prompting researchers to reevaluate the graphite crust.
Additionally, another study also suggested the presence of sulfur in Mercury’s iron core. The presence of sulfur could have an effect on the crystallization of Mercury’s magma ocean, which challenges the initial claim that only graphite was present during this phase.
Recreating the conditions of Mercury’s interior
To recreate the conditions of Mercury’s interior, the researchers used a combination of high-pressure and temperature experiments and thermodynamic modeling.
“We use the large-volume press to mimic the high-temperature, high-pressure conditions of Mercury’s core-mantle boundary and combine them with geophysical models and thermodynamic calculations,” Dr. Lin explained.
They used synthetic silicate as a starting material to reproduce the composition of Mercury’s mantle, a method commonly used to study the interiors of planets.
The researchers reached pressure levels of up to 7 gigapascals (GPa), about seven times the pressure found in the deepest parts of the Mariana Trench.
Under these conditions, the team studied how minerals (those present inside Mercury) melt and reach equilibrium phases and characterized these phases, focusing on those of graphite and diamond.
They also analyzed the chemical composition of the experimental samples.
“In the lab, we try to replicate the extreme pressures and temperatures of a planet’s interior. This is sometimes challenging because you have to tailor the devices to the needs. The experimental setups have to be extremely precise to simulate these conditions,” says Dr. Lin.
They also used geophysical modeling to study observed data on Mercury’s interior.
“Geophysical models come mainly from data collected by spacecraft and tell us the fundamental structures of a planet’s interior,” Dr. Lin said.
They used the model to predict phase stability, calculate CMB pressures and temperatures, and simulate the stability of graphite and diamond under extreme temperatures and pressures.
Diamonds are formed under pressure
By integrating experimental data with geophysical simulations, the researchers were able to estimate Mercury’s CMB pressure to be about 5.575 GPa.
With a sulfur content of about 11%, the researchers observed a considerable temperature variation of 358 Kelvin in Mercury’s magma ocean. The researchers suggest that while graphite was likely the dominant carbon phase during crystallization of the magma ocean, crystallization of the core led to the formation of a diamond layer at the CMB.
“Sulfur lowers the liquidus of Mercury’s magma ocean. If diamond forms in the magma ocean, it can sink to the bottom and be deposited in the CMB. On the other hand, sulfur also contributes to the formation of an iron sulfide layer in the CMB, which is related to the carbon content during planetary differentiation,” Dr. Lin explained.
Planetary differentiation refers to the process by which a planet becomes structured internally, i.e. the center or core, toward which heavier minerals sink, and the surface or crust, toward which lighter minerals rise.
According to their findings, the CMB’s diamond layer is estimated to be between 15 and 18 kilometers thick. They also suggest that the current temperature of Mercury’s CMB is close to the point where graphite can transform into diamond, which would stabilize the CMB’s temperature.
Carbon-rich exoplanetary systems
One implication of these findings concerns Mercury’s magnetic field, which is abnormally strong for its size.
Dr. Lin explained: “The carbon in the molten core becomes supersaturated as it cools, forming diamond and floating toward the CMB. The high thermal conductivity of diamond helps efficiently transfer heat from the core to the mantle, causing temperature stratification and convection change in Mercury’s liquid outer core, thereby affecting the generation of its magnetic field.”
In simpler terms, as heat is transferred from the core to the mantle, it influences temperature gradients and convection in Mercury’s liquid outer core, which affects its magnetic field generation.
Dr. Lin also highlighted the crucial role played by carbon in the formation of carbon-rich exoplanetary systems.
“This could also be relevant for understanding other terrestrial planets, particularly those with similar sizes and compositions. The processes that led to the formation of a diamond layer on Mercury could also have occurred on other planets, potentially leaving similar signatures,” Dr. Lin concluded.
More information:
Yongjiang Xu et al., A core-mantle boundary containing diamonds on Mercury, Nature Communications (2024). DOI: 10.1038/s41467-024-49305-x.
© 2024 Science X Network
Quote:Modeling study suggests diamond layer at core-mantle boundary on Mercury (2024, July 10) retrieved July 10, 2024 from https://phys.org/news/2024-07-diamond-layer-core-mantle-boundary.html
This document is subject to copyright. Apart from any fair dealing for the purpose of private study or research, no part may be reproduced without written permission. The content is provided for informational purposes only.