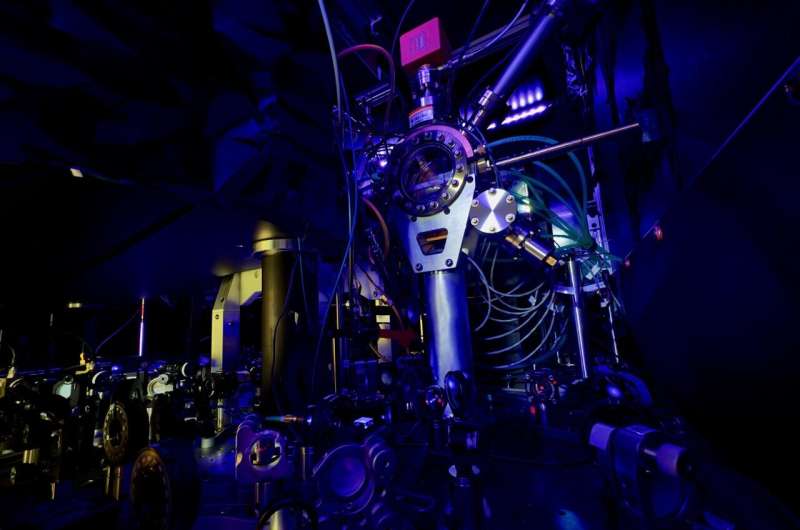
An image of the larger system, showing the optics, vacuum components and control systems needed to create the most accurate clock. Credit: Kyungtae Kim at JILA
Researchers from the Ye Lab at JILA (the National Institute of Standards and Technology and the University of Colorado at Boulder) and the University of Delaware have recently created a high-precision optical lattice clock based on trapped strontium atoms. Their clock, presented in a Physical Exam Letters paper, has a total systematic uncertainty of 8.1 x 10-19which represents the lowest uncertainty reported to date.
“This paper is the culmination of decades of work in the Ye lab to build the best clocks,” Alexander Aeppli, a co-author of the paper, told Phys.org. “Measuring time is a fundamental task in physics, and every advance in the precision and accuracy of measurements opens the door to studying new phenomena and creating new technologies.”
Most existing time-measuring technologies specifically measure the period during which an electron oscillates in a cesium atom. These instruments are known as “microwave atomic clocks” because the frequencies of the oscillations they measure are in the microwave band, resembling the frequencies of electromagnetic oscillations inside a microwave oven.
“Many recent atomic clocks, including ours, use an ‘optical’ transition, where the frequency of the oscillation is similar to the frequency of visible light,” Aeppli explains. “Using a much higher frequency is like using a ruler with finer graduations, subdividing an extra second and immediately allowing for more accurate timekeeping.”
Much of the recent research at JILA’s Ye Lab has focused on developing atomic clocks that can measure time with high precision. The latest study by Aeppli and colleagues builds on progress at the Ye Lab and other institutes around the world that has demonstrated the potential for building precise optical lattice clocks using strontium atoms.
“A classic clock is made up of three parts: an oscillator, a counter, and a reference,” Aeppli explains. “In a classic pendulum clock, the oscillator is a pendulum that swings back and forth once per second. A set of gears counts this movement and advances the second, minute, and hour hands. Finally, the reference is the position of the sun in the sky, which is noon when the sun is directly overhead.”
Optical lattice clocks operate on the same three principles as conventional clocks. However, in this type of clock, the oscillator, counter, and frequency reference take a very different form.
In optical lattice clocks, the oscillator is an ultra-stable laser, while the counter is a frequency comb (i.e., an instrument for measuring optical frequencies by recording the repetition rate of a continuous sequence of light pulses). The frequency reference, in turn, is made of trapped atoms, which in the team’s clock are specifically strontium atoms.
“The frequency comb is stabilized with respect to the laser, and the laser is stabilized with respect to a specific electronic transition in the strontium atoms,” Aeppli said.
“Every few seconds, we shine the laser on the atoms for 2.4 seconds. If the laser drifts off the atomic resonance, we correct for that drift. The frequency comb converts optical frequencies to microwave frequencies, and the beauty of this device is that the frequency stability of the microwave frequency is the same as the optical frequencies.”
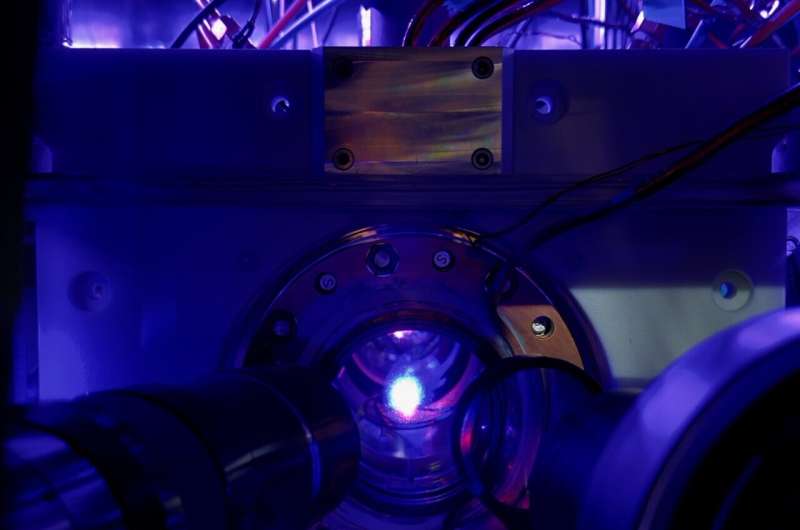
Image of trapped strontium atoms fluorescing under blue light. The atoms are trapped in an ultra-high vacuum before being probed by the clock’s laser to determine the transition frequency. Credit: Kyungtae Kim at JILA.
Once the frequency comb converts the optical frequencies to microwave frequencies, the periods of these frequencies are analyzed by simple electronic means to obtain a second, which is fundamentally related to the frequency of the strontium transition. In the clock developed by Aeppli and his colleagues, strontium atoms are trapped in a standing light wave produced by two mirrors.
“Just like a lattice, the atoms are confined to this light in a periodic manner, with a group of a few atoms located every 0.5 um,” Aeppli explained.
“This technology allows us to trap a hundred thousand strontium atoms at a time, which means that each time we make a measurement of the strontium transition frequency, we can measure many atoms simultaneously, giving a very precise measurement. This contrasts with optical ion clocks which use an electronic transition within a single trapped ion, meaning each measurement is much noisier.”
Although optical lattice clocks have already demonstrated their ability to tell time with exceptional accuracy, they can also have limitations. In particular, their underlying design based on light trapping can affect their accuracy, as it can shift the transition frequency.
Compared to previously proposed optical lattice clocks, the clock introduced by Aeppli and colleagues uses lower intensity light trapping, which significantly increases its accuracy.
“Most of the results obtained in this work are technical,” Aeppli said. “One of the biggest changes in strontium optical lattice clocks comes from interactions with thermal emission from the surrounding medium. We are now better able to characterize this effect and understand how it shifts the natural transition frequency of the strontium atom.”
The work of this team of researchers highlights the enormous potential of optical lattice clocks based on strontium atoms, suggesting that they could perhaps even contribute to the redefinition of the SI second. Aeppli and his colleagues hope that their results will inform future studies in this field, paving the way for the development of increasingly precise clocks.
“On a larger scale, we hope our work shows that there is a continuous path toward making more accurate clocks, and we have not yet seen a fundamental limit to clock accuracy,” Aeppli said.
“Although we have built a clock with excellent accuracy, we have not yet used it to tell time. Still, it is important to compare clocks to understand their limitations. As in the past, we are currently working with colleagues at the National Institute of Standards and Technology (NIST) to compare their platforms.”
Ye Lab researchers are currently conducting a variety of other clock experiments, each aimed at designing approaches to building more advanced atomic clocks.
Two promising approaches they are exploring involve using quantum entanglement to reduce the noise associated with measuring the transition frequency and using a nuclear transition, which can give even better precision.
More information:
Alexander Aeppli et al, Clock with 8×10−19 Systematic uncertainty, Physical Exam Letters (2024). DOI: 10.1103/PhysRevLett.133.023401. On arXiv: DOI: 10.48550/arxiv.2403.10664
© 2024 Science X Network
Quote:Optical lattice clock based on strontium atoms achieves unprecedented precision (2024, July 24) retrieved July 24, 2024 from https://phys.org/news/2024-07-optical-lattice-clock-based-strontium.html
This document is subject to copyright. Apart from any fair dealing for the purpose of private study or research, no part may be reproduced without written permission. The content is provided for informational purposes only.