Summary: A new study reveals that the epigenetic state of neurons determines their role in memory formation. Neurons with open chromatin are more likely to be recruited into memory traces, showing higher electrical activity during learning.
Researchers have shown that manipulating these epigenetic states in mice can enhance or impair learning. This discovery shifts the focus from synaptic plasticity to nuclear processes, offering potential new avenues for treating cognitive disorders.
Highlights:
- Neurons with open chromatin states are more likely to be involved in memory formation.
- Manipulating the epigenetic state of neurons in mice can enhance or impair learning.
- This research shifts the focus from synaptic plasticity to nuclear processes in learning.
Source: EPFL
When we create a new memory, the brain undergoes physical and functional changes known as a “memory trace.” A memory trace represents the specific patterns of activity and structural changes in neurons that occur when a memory is formed and later recalled.
But how does the brain “decide” which neurons will be involved in a memory trace? Studies have suggested that the inherent excitability of neurons plays a role, but the currently accepted view of learning has neglected to look inside the control center of the neuron itself, its nucleus. Within the nucleus, there seems to be a whole other dimension that has not been explored: epigenetics.
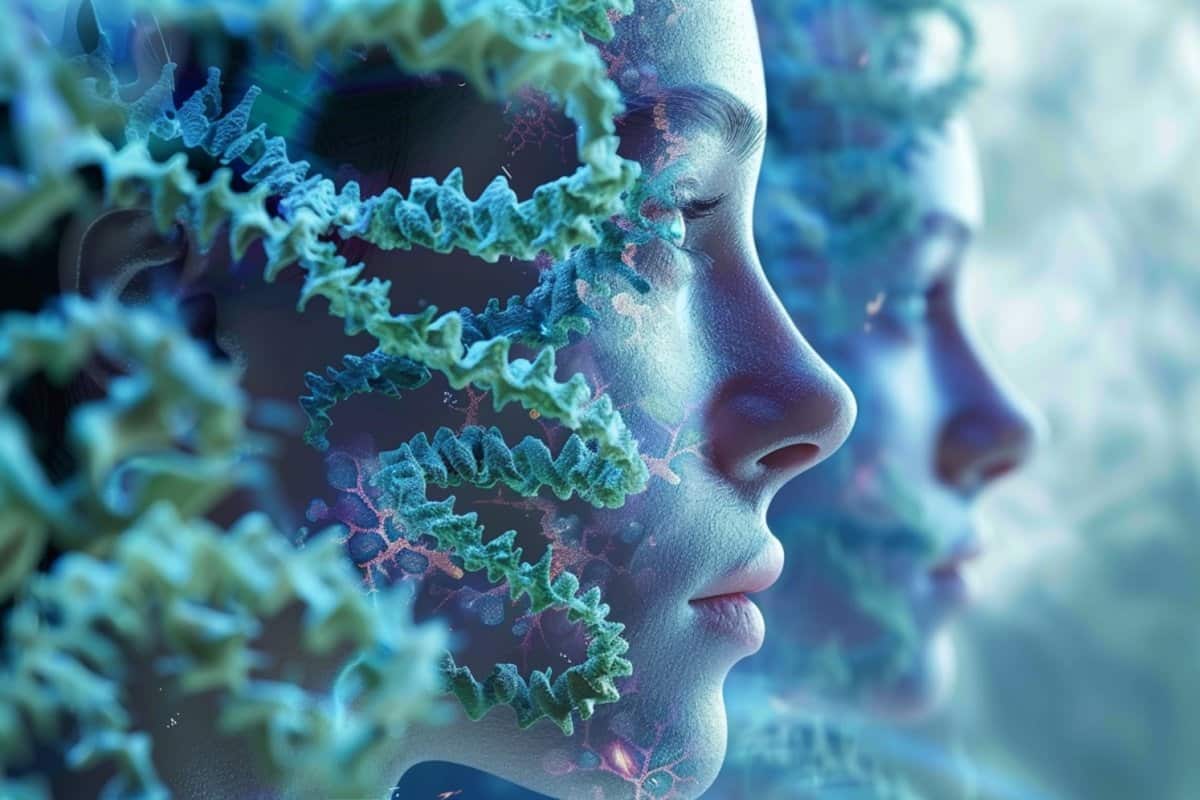
In every cell of a living organism, the genetic material encoded by DNA is the same, but the different types of cells that make up the body, such as skin cells, kidney cells, or nerve cells, each express a different set of genes. Epigenetics is the mechanism by which cells control the activity of these genes without changing the DNA sequence.
EPFL scientists led by neuroscientist Johannes Gräff have now investigated whether epigenetics could affect the likelihood that neurons are selected for memory formation.
Their research on mice, now published in Scienceshows that the epigenetic state of a neuron is essential to its role in memory encoding.
“We are shedding light on the first step in memory formation at a DNA-centric level,” Gräff says.
Gräff and his team wondered whether epigenetic factors could influence a neuron’s “mnemonic” function. A neuron can be epigenetically open when the DNA inside its nucleus is untangled or loose; and closed when the DNA is compact and tight.
They found that it was the open neurons that were most likely to be recruited into the “memory trace,” the sparse collection of neurons in the brain that exhibit electrical activity when learning something new. Indeed, neurons with more open chromatin were also those that exhibited higher electrical activity.
The EPFL scientists then used a virus to deliver epigenetic enzymes to artificially induce the neurons to open. They found that the corresponding mice learned much better. When the scientists used the opposite approach to shut down the neurons’ DNA, the mice’s ability to learn was negated.
These results open up new avenues for understanding learning within the neuron’s nucleus and could one day even lead to the development of drugs to enhance learning. As Gräff explains: “They move away from the dominant neuroscientific view of learning and memory, which emphasizes the importance of synaptic plasticity, and now focus on what happens inside the nucleus of a neuron, on its DNA.”
“This is particularly important because many cognitive disorders such as Alzheimer’s disease and post-traumatic stress disorder are characterized by defective epigenetic mechanisms.”
About this news on memory and epigenetics research
Author: Nik Papageorgiou
Source: EPFL
Contact: Nik Papageorgiou – EPFL
Picture: Image credited to Neuroscience News
Original research: Access closed.
“Chromatin plasticity predetermines neuronal eligibility for memory trace formation” by Johannes Gräff et al. Science
Abstract
Chromatin plasticity predetermines neuronal eligibility for memory trace formation
INTRODUCTION
During development, epigenetic heterogeneity gives rise to different cell types with different functions. By stably ordering the activation and deactivation of genomic loci to catalyze specific signaling cascades, epigenetic mechanisms play a critical role in lineage commitment and cell differentiation. However, it remains unclear whether chromatin plasticity plays a similarly important role in the development of dynamic functions in fully differentiated cells, such as adult neurons.
One of the most fascinating features of neurons is their ability to encode information. Indeed, for each new piece of information stored, the brain deploys only a subset of its neurons, which implies that even within the same developmentally defined cell type, not all neurons are equally adept at encoding information at any given time.
REASONING
The dependence of memory formation on neuronal selection led us to ask whether chromatin architecture could be sufficiently heterogeneous, among seemingly homogeneous cellular identities, to drive information coding. And more specifically, whether increased chromatin plasticity could be a catalytic force to prepare neurons to be preferentially selected for memory formation.
RESULTS
Focusing on the mouse lateral amygdala, a key brain region responsible for encoding associative forms of memory, we found that its excitatory neurons indeed exhibit heterogeneous chromatin plasticity and, moreover, that those preferentially recruited to learning-activated neurons were enriched in hyperacetylated histones, an epigenetic modification abundant in the brain.
To functionally test this correlation between chromatin plasticity and information coding, we then manipulated histone acetylation levels by increasing or decreasing histone acetyltransferases (HATs) in these neurons. We found that a gain-of-function of histone acetylation-mediated epigenetic plasticity facilitated neuronal recruitment to the memory trace while a loss-of-function of histone acetylation prevented memory allocation.
Interested in the molecular mechanisms underlying this selection, we then performed single-nuclear multiome sequencing for the simultaneous assessment of chromatin accessibility and gene expression changes occurring in epigenetically modified neurons.
These results revealed increased chromatin accessibility or expression at genomic locations closely related to structural and synaptic plasticity, as well as neuronal excitability, which has been identified as an important physiological process for information coding. Accordingly, we found that increased chromatin plasticity also led to increased intrinsic neuronal excitability and promoted structural and functional synaptic remodeling.
For a process to truly qualify as an influencer of memory allocation, it must also promote memory retention. To this end, we tested HAT-injected mice on Pavlovian fear conditioning, a type of associative memory, and found that they exhibited significantly stronger fear memory—an effect that lasted for up to eight days. Notably, optogenetic silencing of the epigenetically altered neurons prevented fear memory recall, suggesting a cell-autonomous relationship between chromatin plasticity and memory trace formation.
Finally, by combining Förster resonance energy transfer (FRET) tools and calcium imaging in individual neurons, we revealed that the link between chromatin plasticity and intrinsic neuronal excitability occurs endogenously, cell-autonomously, and in real time.
CONCLUSION
Our results show that the eligibility of a neuron to be recruited into the memory trace depends on its epigenetic state before learning, and thus identify chromatin plasticity as a new form of plasticity important for information encoding. The epigenetic landscape of a neuron could therefore represent an adaptable model to record and integrate environmental signals in a dynamic, yet sustainable, manner.