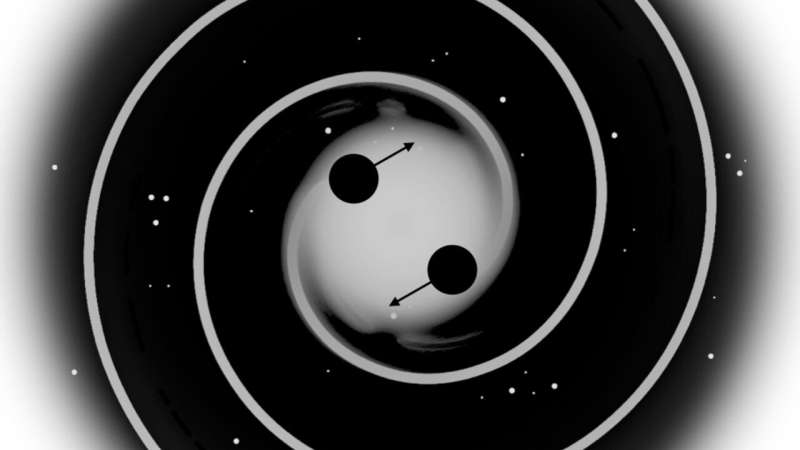
Illustrative representation of a black hole binary immersed in a spike of dark matter at the center of a galaxy. Credit: Original image: NASA science.nasa.gov/resource/spiral-galaxy-blue/. Modified by Alvarez, Cline, and Dewar.
In a new study, Canadian scientists have proposed a solution to the final parsec problem of supermassive black hole (SMBH) mergers using self-interacting dark matter.
When two galaxies merge, gas and dust collide, leading to the formation of stars. However, the stars themselves do not collide due to their large distances. The SMBHs at the centers of the two galaxies also begin to merge.
However, the merger of black holes stops when they are separated by 1 parsec (or 30.9 trillion kilometers). This problem is known as the “final parsec problem” in astronomy and astrophysics.
The study, published in Physical Review Letters (PRL)attempts to solve this problem and explain the gravitational wave spectrum observed in 2021 by the Pulsar Timing Array collaborations.
Phys.org spoke with the study’s first author, Dr. Gonzalo Alonso Alvarez, a postdoctoral fellow at the University of Toronto.
“What struck us most when the Pulsar Timing Array collaborations announced evidence for a gravitational wave spectrum was that there was room to test new particle physics scenarios, particularly dark matter self-interactions, even within the standard astrophysical explanation of supermassive black hole mergers,” he said.
Why stop at 1 parsec?
When the SMBHs of two merging galaxies are separated by one parsec, two contradictory phenomena come into play.
First, large objects like an SMBH cause ripples in spacetime, which leads to the formation of gravitational waves that propagate throughout spacetime. These gravitational waves carry energy away from the source. When two SMBHs merge, the gravitational waves carry energy away from the merger, causing the black holes to spiral inward faster.
The second force is a frictional force called dynamic friction. When massive objects like black holes pass through a medium (like dust and stars), they leave behind a trail of disturbed fluid called a wake. For example, when a ship moves through water, it leaves behind a trail of turbulent water: this is its wake.
Particles attracted to the SMBH by gravity can cause a drag force, which is dynamic friction. This friction opposes the motion of the massive object, forcing it to slow down. In the case of two SMBHs merging, this can prevent them from moving closer to each other.
“Previous calculations have shown that this process stops when the black holes are about 1 parsec apart, a situation sometimes called the final parsec problem,” Dr. Alvarez explained.
This is where dynamic friction comes into play, which can either oppose or promote the fusion of the two SMBHs.
Self-interactive dark matter
Researchers suggest that a form of dark matter could be the solution to this problem.
“In this paper, we show that including the previously neglected effect of dark matter can help black holes overcome this final parsec of separation and merger, thereby emitting a gravitational wave signal that matches that observed by the Pulsar Timing Arrays,” said Dr. Alvarez.
In a galaxy, dark matter is mainly present in the galactic halo, the region surrounding the visible galaxy. But it is also present near the galactic core, where the SMBH is located. Therefore, the nature of dark matter could play a crucial role in the merger of SMBHs.
Self-interacting dark matter (SIDM) is a hypothetical form of dark matter in which dark matter particles interact with each other via a new, unknown force.
In galaxies containing SIDM, interactions between dark matter particles can affect the density (distribution) and velocity of dark matter, leading to a more efficient funneling of matter and energy towards the SMBH, which could potentially overcome dynamical friction.
A delicate balance
To explore the role of SIDM in the SMBH merger, the researchers performed detailed calculations of dark matter density profiles around SMBHs for SIDM and cold (less interactive) dark matter.
They also modeled dynamical friction effects on SMBH orbits, calculated energy transfer between SMBH and dark matter, and performed simulations of gravitational wave spectra under different dark matter scenarios.
They then compared these results with observational data from pulsar timing networks.
The researchers found that the interaction cross section of dark matter particles must be within an optimal range. A larger cross section, which means more frequent interactions, causes dark matter particles to interact and disperse, flattening the density profile near SMBHs.
This reduction in density decreases the dynamic friction required for SMBH fusion.
“On the other hand, sufficiently frequent dark matter self-interactions are required to prevent this profile from being scattered by the black hole’s motion,” explains Dr. Alvarez.
The ideal cross-section range allows enough interactions to influence the motion of SMBHs without over-dispersing the dark matter, thus maintaining sufficient dynamic friction to facilitate the fusion process.
The researchers found that this value was between 2.5 and 25 cm.2/g. This means that for every gram of dark matter, the effective surface area on which the particles interact should be between 2.5 and 25 square centimeters.
Rate-dependent interactions
The researchers also found that the speed of the SIDM particles must be optimal. This speed is in turn influenced by the mass of the unknown force carrier or mediator, which facilitates the interaction between the SIDM particles.
If the mediator is heavy, this could mean that dark matter particles only interact significantly when they are moving slowly relative to each other. Conversely, if the mediator is light, interactions could occur at higher speeds.
“Interestingly, this velocity dependence is theoretically well motivated. This is precisely what we find if the particle that acts as the force carrier for dark matter self-interactions has a mass that is about 1 percent of the mass of the dark matter particle,” Dr. Alvarez said.
The researchers estimated this value to be between 300 and 600 km/s.
“These velocity-dependent self-interactions leave an imprint on the gravitational wave spectrum because when black holes are within a fraction of a parsec of each other, a significant fraction of the orbital energy is lost to dark matter friction rather than gravitational wave emission, thereby relatively suppressing the gravitational wave signal at some frequencies compared to others,” added Dr. Alvarez.
Implications and future work
The researchers’ SIDM particle model predicted that gravitational waves would be weaker or less intense at lower frequencies. This prediction matched what was observed in the real data.
They also showed that SIDM with velocity-dependent cross section can solve the final parsec problem and survive the fusion process.
“We found that the evolution of the black hole orbit is very sensitive to the microphysics of dark matter, which means we can use the gravitational wave emission from SMBH binaries to constrain dark matter models,” said Dr. Alvarez, discussing the impact of their work. “This provides a new window to probe the nature of dark matter in the deepest regions of galaxies that were previously inaccessible observationally.”
The team is also refining its model and developing numerical simulations to confirm the results found in this study. These simulations will provide a better understanding of how dark matter profiles respond to the energy injected by merging black holes.
More information:
Gonzalo Alonso-Álvarez et al., Self-interacting dark matter solves the final parsec problem of supermassive black hole mergers, Physical Exam Letters (2024). DOI: 10.1103/PhysRevLett.133.021401.
© 2024 Science X Network
Quote:New study uses self-interacting dark matter to solve the final parsec problem (2024, July 30) retrieved July 30, 2024 from https://phys.org/news/2024-07-interacting-dark-parsec-problem.html
This document is subject to copyright. Apart from any fair dealing for the purpose of private study or research, no part may be reproduced without written permission. The content is provided for informational purposes only.