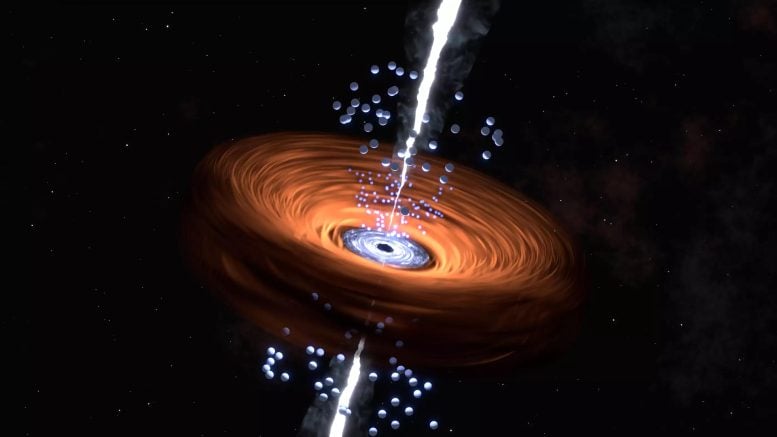
Artist’s impression of the bright central region of a quasar, an active galaxy. The supermassive black hole at the center is surrounded by a bright disk of gas and dust. The dust further out can obscure the view of the interior and shines mainly in the mid-infrared range, light that can be analyzed by the James Webb Space Telescope. A beam of clustered, high-energy particles shoots out into space from the immediate vicinity of the black hole perpendicular to the disk. Credit: © T. Müller / MPIA
Surprisingly unspectacular: the black hole already weighed more than a billion solar masses in the early universe, despite an average appetite.
Looking at the early stages of the 13.8 billion year old universe,
” data-gt-translate-attributes=”({“attribute”:”data-cmtooltip”, “format”:”html”})” tabindex=”0″ role=”link”>James Webb Space Telescope spotted a galaxy as it existed only 700 million years after the
” data-gt-translate-attributes=”({“attribute”:”data-cmtooltip”, “format”:”html”})” tabindex=”0″ role=”link”>Big BangIt is amazing how the
” data-gt-translate-attributes=”({“attribute”:”data-cmtooltip”, “format”:”html”})” tabindex=”0″ role=”link”>black hole At its center, the core could already weigh a billion solar masses when the universe was still in its infancy. James Webb’s observations were intended to take a closer look at the power mechanism, but they revealed nothing extraordinary. Apparently, black holes were already developing in the same way as they do today. But the result is all the more significant: it could show that astronomers know less than they thought about the formation of galaxies. And yet, the measurements are by no means disappointing. On the contrary.
The mystery of the first black holes
The first billion years of cosmic history pose a challenge: The first known black holes at the centers of galaxies had surprisingly large masses. How did they become so massive, so quickly? The new observations described here provide strong evidence against some proposed explanations, including an “ultra-efficient power supply” for the first black holes.
The limits to the growth of supermassive black holes
Stars and galaxies have changed enormously over the past 13.8 billion years, the lifetime of the Universe. Galaxies have grown larger and gained more mass, either by consuming surrounding gas or (occasionally) by merging with each other. For a long time, astronomers assumed that the supermassive black holes at the centers of galaxies gradually grew, along with the galaxies themselves.
But a black hole can’t grow arbitrarily fast. Matter falling onto a black hole forms a swirling, hot, glowing “accretion disk.” When this happens around a supermassive black hole, the result is an active galactic nucleus. The brightest such objects, called quasars, are among the brightest astronomical objects in the entire cosmos. But this brightness limits the amount of matter that can fall onto the black hole: Light exerts pressure that can prevent additional matter from falling in.
How did black holes become so massive and so fast?
This is why astronomers have been surprised to see, over the past twenty years, observations of distant quasars reveal very young black holes, whose masses reached 10 billion solar masses. Light takes time to propagate from a distant object to us. Observing distant objects is therefore like diving into the distant past. We see the most distant quasars known as they were at a time called the “cosmic dawn”, less than a billion years after the Big Bang, when the first stars and galaxies formed.
Explaining the formation of these early massive black holes poses a significant challenge to current models of galaxy evolution. Could it be that early black holes were much more efficient at accreting gas than their modern counterparts? Or could the presence of dust affect quasar mass estimates in a way that led researchers to overestimate the mass of early black holes? Many explanations have been proposed to date, but none are widely accepted.
A closer look at early black hole growth
Determining which explanation is correct requires a more complete picture of quasars than has been available to date. With the advent of the JWST space telescope, and especially its mid-infrared MIRI instrument, astronomers’ ability to study distant quasars has taken a giant leap forward. At measuring the spectra of distant quasars, MIRI is 4,000 times more sensitive than any previous instrument.
Instruments like MIRI are built by international consortia, in which scientists, engineers, and technicians work closely together. Naturally, a consortium has a strong interest in checking whether its instrument works as well as expected. In exchange for building the instrument, consortia are usually given a certain amount of observing time. In 2019, years before JWST was launched, the European MIRI consortium decided to use some of that time to observe what was then the most distant quasar known, an object designated J1120+0641.
Observation of one of the first black holes
Analysis of the observations was entrusted to Sarah Bosman, a postdoctoral researcher at the Max Planck Institute for Astronomy (MPIA) and a member of the European MIRI consortium. MPIA’s contributions to the MIRI instrument include the construction of a number of essential internal parts. Bosman was invited to join the MIRI collaboration specifically to provide her expertise on how best to use the instrument to study the early Universe, particularly the first supermassive black holes.
The observations were made in January 2023, during the first JWST observation cycle, and lasted about two and a half hours. They are the first mid-infrared study of a quasar at cosmic dawn, just 770 million years after the Big Bang (redshift z = 7). The information comes not from an image, but from a spectrum: the rainbow decomposition of the object’s light into components of different wavelengths.
Tracking fast moving dust and gases
The general shape of the mid-infrared spectrum (“continuum”) encodes the properties of a large dust torus that surrounds the accretion disk of typical quasars. This torus helps guide matter toward the accretion disk, “feeding” the black hole. The bad news for those who prefer alternative rapid growth modes to primitive massive black holes: the torus, and by extension the feeding mechanism for this very early quasar, appears to be the same as for its more modern counterparts. The only difference is one that no model of rapid growth of early quasars had predicted: a slightly higher dust temperature, about a hundred kelvins, than the 1,300 K found for the hottest dust of less distant quasars.
The shorter wavelength part of the spectrum, dominated by emissions from the accretion disk itself, shows that for us, as distant observers, the quasar light is not attenuated by a larger than usual amount of dust. Arguments that we may simply be overestimating the mass of primitive black holes because of the extra dust are also not the answer.
First ‘surprisingly normal’ quasars
The quasar’s broad-line region, where clumps of gas orbit the black hole at speeds close to the speed of light – from which the mass of the black hole, density and ionization of the surrounding matter can be inferred – also appears normal. By almost all properties inferred from the spectrum, J1120+0641 is no different from quasars of later epochs.
“Overall, the new observations only add to the mystery: the first quasars were surprisingly normal. No matter what wavelength we observe them at, quasars are almost identical at all epochs of the Universe,” says Bosman. Not only the supermassive black holes themselves, but also their feeding mechanisms were apparently already fully “mature” when the Universe was only 5% of its current age. Ruling out a number of alternative solutions, the results strongly support the idea that supermassive black holes started out with considerable masses from the start, in the jargon of astronomy: that they are “primordial” or “mass-seeded.” Supermassive black holes did not form from the remains of primitive stars, but then became massive very quickly. They must have formed very early with initial masses of at least a hundred thousand solar masses, probably by the collapse of primitive massive gas clouds.
Reference: “A mature quasar at cosmic dawn revealed by JWST rest-frame infrared spectroscopy” by Sarah EI Bosman, Javier Álvarez-Márquez, Luis Colina, Fabian Walter, Almudena Alonso-Herrero, Martin J. Ward, Göran Östlin, Thomas R. Greve, Gillian Wright, Arjan Bik, Leindert Boogaard, Karina Caputi, Luca Costantin, Andreas Eckart, Macarena García-Marín, Steven Gillman, Jens Hjorth, Edoardo Iani, Olivier Ilbert, Iris Jermann, Alvaro Labiano, Danial Langeroodi, Florian Peilußker, Pierluigiker Rinaldi, Martin Topinka, Paul van der Werf, Manuel Güdel, Thomas Henning, Pierre-Olivier Lagage, Tom P. Ray, Ewine F. van Dishoeck and Bart Vandenbussche, June 17, 2024, Astronomy of nature.
DOI: 10.1038/s41550-024-02273-0